Sharon Glotzer Probing the What-Ifs with Statistical Mechanics
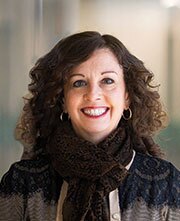
Sharon Glotzer. Photo courtesy of Glotzer.
If you find quantum mysteries and astrophysical wonders far more captivating than statistical mechanics, spend a few minutes talking to Sharon Glotzer. You might change your mind.
“Statistical mechanics is incredibly powerful,” Glotzer says. “If you tell me that you have a beaker of water containing a bunch of gold nanoparticles, without knowing too much about it I can predict whether the nanoparticles are likely to stay in the water, precipitate out, or self-assemble into some ordered structure.”
But to understand a system in classical or quantum mechanics, you need all the details. In classical mechanics, she explains, you need to solve Newton’s equations of motion, track what every single object is doing at every moment, and know what forces are acting on every object and how that affects all the other objects. Things get “really complicated really fast,” she says.
In statistical mechanics, it doesn’t even matter if the objects are atoms, nanoparticles, granular particles, tiny robots, self-propelled bacteria, or even birds. If a system is ergodic—meaning that it can, in principle, explore all available configurations (or microstates)—you can predict its collective behavior with statistical mechanics, Glotzer explains. Studying systems this way often reveals universalities or commonalities across different systems, scales, and phenomena.
The practical applications of statistical mechanics research are central to industries ranging from cosmetics to semiconductors, but Glotzer is most intrigued by the what-ifs. They’re the focus of her research group at the University of Michigan, where she’s chair of the chemical engineering department.
Using computer models, Glotzer’s group looks at systems governed by statistical mechanics and then changes the rules or creates entirely new systems that don’t exist yet, but could someday, to see what happens. It’s kind of like playing God, she says. “You can say, ‘These are my objects, and these are the rules by which they will interact with one another—show me what happens under this condition.’” If the code doesn’t exist to do the desired simulation, her group writes it―and then makes it available for others to explore.
Researchers in her group can do millions of computer experiments in a fraction of the time it would take to do one of them in a lab. It’s a way of scoping out research areas, guiding collaborators and the experimental community to where they might find the most exciting, intriguing, or useful results.
In June of 2022, Glotzer was awarded a Vannevar Bush Faculty Fellowship from the US Department of Defense, her second. These high-profile, single-investigator awards of up to $3 million support blue-sky research―research that doesn’t map immediately to a real-world application but is likely to have transformative potential down the road.
Glotzer’s fellowship research explores the notion that entropy sometimes acts like a chemical bond. That might sound a little strange. Entropy is a system quantity, as Glotzer freely admits. It doesn’t make sense to talk about the entropy at a specific point in space, as you might a chemical bond. But it’s sometimes useful to consider the effects of entropy at a local level, she says.
Glotzer gives the following example. Many nanoparticles are actually nanocrystals, shaped like multifaceted gemstones. These nanoparticles can self-assemble into even larger crystals. Her group discovered that in the absence of any interactions between these nanoparticles and with just one constraint―they can’t overlap―a surprisingly diverse set of complicated crystals can emerge. “And it turns out that in those systems, when you have no explicit interaction energy, the only driving force is entropy,” she says.
To explain how these particles organize locally, Glotzer and her team formulated a theory of entropic bonding. And they’re using the Bush fellowship to explore what that theory reveals about how entropy works, why atoms and nanoparticles can both form crystals of such great complexity, and all the ways you can potentially mix and match atoms or nanoparticles to get crystal structures. “We’re hoping that the theory of entropic bonding can even help us understand what happens when you have lots of forces between atoms or molecules,” Glotzer says. The exploration could potentially change the way people think about—and teach—basic chemistry.
Although she’s chair of the chemical engineering department, Glotzer is a proud physicist. She was inducted into Sigma Pi Sigma as an undergraduate physics major at the University of California, Los Angeles, and earned a PhD in physics at Boston University. After graduating, she worked at the National Institute of Standards and Technology (NIST) in Maryland, eventually cofounding and directing the NIST Center for Theoretical and Computational Materials Science before moving to the University of Michigan as a professor.
“I work with PhD students who come from chemical engineering, chemistry, physics, material science, macromolecular science and engineering, computer science, and mechanical engineering―all of these different disciplines,” Glotzer says. “Physics underlies all of it.” If she had to go back in time, she’d still major in physics. That’s because of the subject matter and because “physicists learn how to see past all the tiny details that make different systems different, and see instead how they are the same.”
Glotzer receives so many invitations to give lectures and seminars that she can’t possibly accept them all. But she always says yes to those that come from graduate students. It brings back the excitement of being a student and meeting the authors of papers who inspired her own curiosity and research trajectory, she says. “I’m still having so much fun doing science, just like when I was a student.”
To hear what sparked Sharon Glotzer’s interest in science and learn more about her research, check out her 2021 interview with Quanta magazine in their podcast, The Joy of X. www.quantamagazine.org/sharon-glotzers-deep-curiosity-about-order-from-c...
Is there a Sigma Pi Sigma member you’d like to see featured in a Member Spotlight? Let us know at sps [at] aip.org.