NASA Mission Probes Earth’s Magnetic Defenses
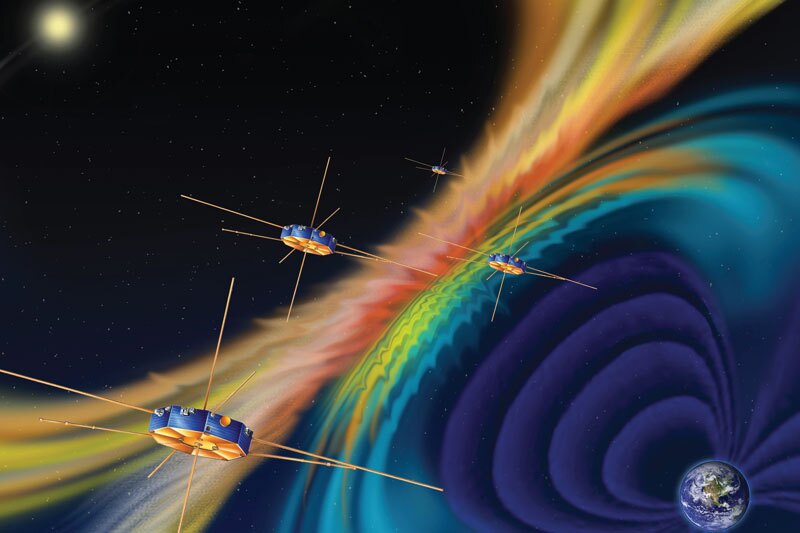
Artist rendition of MMS spacecraft. MMS consists of four identical spacecraft that work together to provide the first three-dimensional view of this fundamental process, which occurs throughout the universe. Image courtesy of NASA.
Have you ever seen a picture of an eclipse showing a beautiful loop at the edge of the Sun? If so, you are seeing particles trapped on a magnetic loop wound out by the bubbling convection taking place on the Sun’s surface. Just as iron filings reveal the field lines of bar magnets, solar prominences reveal the moving magnetic field lines of the Sun.
Sometimes you’ll see one of these prominences explode. That happens when two oppositely-directed fields bump together. They annihilate each other, launching mountains of plasma, which we call coronal mass ejections, out into space, eventually reaching the Earth and causing spectacular auroras and disturbances in the geospace environment known as space weather.
Earth’s own magnetic field protects us from this dangerous radiation and makes life possible on Earth. This field, shaped like a comet, measures about 10 Earth radii (1 Earth radius = 6371 km or 3950 miles) out in front and 30 Earth radii across, with a tail hundreds of Earth radii long. Without it, the solar wind—the supersonic outflow of plasma from the Sun—would sweep away the ionized gases of Earth’s upper atmosphere, resulting in a significant loss of atmospheric material over the history of the planet. That’s what happened on Venus and Mars, neither of which has a global magnetic field.
Our magnetic field is intact but a bit leaky. At the boundary between the magnetic fields of Earth and the Sun, collisions similar to those that occur at the Sun can take place. Those reconnection events can’t be seen but nonetheless are extremely important because they open up holes that allow energy and plasma from the Sun to enter Earth’s vicinity, resulting in space weather.
A 2009 report from the National Academy of Sciences warned of the potential consequences of such geomagnetic storms. Power lines can pick up and transmit ground currents created during these events, and those currents can melt the copper in transformers. The increasing interconnectedness of power grids today makes them increasingly susceptible to widespread outages, such as the one that occurred in Canada in 1989. NASA reported a near miss in July 2012, when a solar superstorm crossed a spot in Earth’s path. Fortunately, our planet wasn’t there at the time.
To better understand the details of reconnection events, on March 12, 2015, NASA launched the four spacecraft that compose the Magnetospheric Multiscale (MMS) mission. Since we can’t see magnetic reconnections happening where the magnetic fields of the Sun and Earth collide, my team is flying our instruments right through them.
I’ve basically been working on similar problems ever since I was a graduate student, but with better and better instruments in different regions of space. My PhD focused on data gathered by instruments placed on the moon by Apollo 14. I was very fortunate to be a part of the twin-spacecraft Dynamics Explorer (DE) mission, the first mission to capture the accelerating electric field that create auroras between one spacecraft at a high altitude and another at a low altitude. At low altitudes we observed downward-directed electrons, and at high altitudes we observed the upgoing ions. We nailed it.
Just as DE did with the auroral acceleration mechanism, MMS is basically trying to nail the physics of reconnection. We’re trying to trap a magnetic reconnection between several spacecraft. We want to measure the curl of the magnetic and electric fields and the plasma during magnetic reconnection. The best way to measure curl is to have four measuring points in a pyramid, so we arranged MMS’s spacecraft in a tetrahedron. This allows us to measure the motion and orientation of the boundaries, and with very sensitive instruments measure locally (not just infer) the electric field along the magnetic field.
What we learn could have implications not just for protecting power grids but for producing power as well. For example, knowing more about reconnection could be important for nuclear fusion. The main reason why nuclear fusion experiments on Earth haven’t been successful to date is because the tokamak reactors work by trapping plasma in a really strong magnetic field. A reconnection event disrupts the field and causes the tokamak to let go of the plasma and dump its energy.
A previous mission I worked on, called Cluster, gave us a good idea of the middle-to-large-scale processes involved in magnetic reconnection. As a charged particle passes from the Sun’s magnetic field to Earth’s, it starts to circle around. The size of the circles it makes depends on the mass of the particle. A proton, for instance, will make circles 2,000 kilometers across. Cluster made measurements on those spatial and temporal scales.
MMS will give us a much finer spatial and temporal resolution, down to 30 milliseconds. Because its spacecraft are closer together than Cluster’s and its time resolution so much better, we can measure changes on the time and space scales of the electron orbits. By observing changes in the electron distribution, we can finally understand the microphysics of reconnection.
The other difference between MMS and Cluster is its orbit. Like a comet, a spacecraft spends most of its time at the apogee of its orbit. MMS’s apogee was optimized to be in the region of Earth’s magnetic field where reconnection is most likely to occur. We hover there, increasing the chance that we will encounter a reconnection event. Cluster went much farther out and spent a lot of time in the solar wind.
By monitoring the Sun, we can forecast changes in space weather that could trigger reconnection with about one to three hours of warning. When something really exciting is coming, we send out an alert email to other researchers saying that an event may occur. The spacecraft can store onboard the most recent four days of data, but can only send down a small fraction of the highest resolution data to the ground. Automatic routines on the spacecraft identify potentially interesting segments of data. Quicklook summaries are sent down, where a “Scientist in the Loop” examines the data and adjusts the on-board algorithm to set quality labels on each segment of data. The data are then transmitted back to earth in priority order by quality, allowing us to be sure we got the best reconnection encounters.
So far there have been a number of events that were very tantalizing. One happened in the magnetic tail in June. We’re analyzing that data now. Then there were two that happened on the side of the magnetosphere when the Sun had several big outbursts in August.
Now we’re heading into the prime part of the mission. Over the next few months, our apogee will be crossing the nose of the magnetosphere on the day side. That’s where most of the action will be. Stay tuned and keep enjoying those wonderful auroras!
To follow our work and learn more about the mission, visit http://mms.gsfc.nasa.gov/