Considering a Career in Physics Research?
Whether you envision working for a college or university, national lab, private company, hospital, or other type of organization, there are a lot of ways to make research a career. It might surprise you to learn that many of these opportunities are outside of physics departments and that, in some cases, you could be competing for jobs with people who have computer science, engineering, or other specialized degrees.
For many undergraduate physics students, especially those in small departments with few faculty, research opportunities on campus are fairly limited. You can expand your exposure to different fields, tools, and techniques by pursuing research experiences and internships both on and off campus, reading about the latest developments in physics, and going to research talks and professional physics meetings. However, it can still be hard to decide what type of research you would like to pursue in graduate school (although not all research jobs require a PhD, many of them do) and as a career.
For example, if you’re interested in studying gravitational waves, you might consider questions like these before approaching potential research advisors. Do you want to study gravitational waves from existing facilities? Work on plans for new gravitational wave observatories? Analyze data? Predict what gravitational wave signals will look like? Characterize or design equipment? Study the causes of gravitational waves? Predict the signals caused by such events? Write computer programs? Work on-site at a detector?
One way to break down the options in a meaningful way is to categorize research approaches as theoretical, experimental, or computational. Researchers with all three approaches often work together closely. Taking stock of your passion, personality, and skills can help you determine which type of research might be the best fit for you during graduate school and beyond.
To give you a better idea of what it means to work in each of these areas, The SPS Observer asked three scientists to share their stories. Since most undergraduate physics departments have at least one experimental physicist on staff, in that category we’ve opted to introduce you to a physicist working in applied physics, an area that falls loosely under the category of experimental physics. Like more traditional experimental physicists, applied physicists tend to be hands-on problem solvers, working regularly with data and instruments. However, by definition they focus on applying physics to real-world situations or technologies. If you would like information on what it means to be a more traditional experimental physicist, consider interviewing one on your campus or at a nearby research lab.

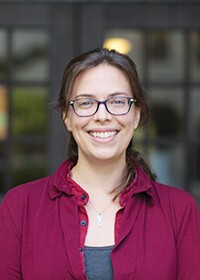
Experimental physicist Jess McIVer. Photo courtesy of Kent Blackburn.
by Jess McIver, Postdoctoral Scholar in Experimental Physics at Caltech
This is excerpted from an article titled “From Theory to Experiment” that first appeared in the Spring 2015 issue of The SPS Observer.
Physics research can usually be classified as theory, experiment, computation, or somewhere in between. Each type of research has its own challenges and rewards.
Theory
Theorists use mathematics and models to explain current phenomena, predict new ones, and describe the laws of the universe. Often these researchers tackle specific problems limited in scope, such as modeling nuanced particle interactions or predicting the amplitude of gravitational waves propagating from shortly after the big bang.
Experiment
Experimentalists test theoretical predictions as well as investigate observable interactions and physical behavior. This generally involves constructing and operating instrumentation used for measurement or observation, on a scale from the rather small (equipment that fits easily inside a small room) to the very large (e.g., the Large Hadron Collider, which has a 27-km circumference). Experimental physics often leads theory, as when a new unpredicted particle is discovered. Likewise, theory often leads experimental activities.
Computation
Computational physics is increasingly becoming a field unto itself. These researchers apply numerical analysis and other computational techniques to physics problems, including large-scale weather simulations, investigations of the properties of semiconductors, or models of protein folding. Computation has deep connections to both theory and experiment.

Computational physicist Rajesh Sathiyanarayanan. Photo by Uma Ramanathan.
by Rajesh Sathiyanarayanan, Technical Staff Member at Applied Materials
I had never seriously considered pursuing a career in physics until halfway through college. In the late 1990s in India, people who were good in math and science mostly went into an engineering track. Fortunately, my college provided the option of double majoring, an unusual opportunity in India, so I ended up studying physics and computer science. This naturally paved a way for me to go into the field of computational physics later. Although I was initially inclined towards working on theoretical cosmology in graduate school, funding scenarios and job prospects in that field made me change my mind. After talking to faculty members from different research groups, I decided to specialize in computational materials science.
Broadly speaking, computational physicists use simulations to provide a reasonably accurate description of systems that are either too complex for a purely theoretical treatment or require considerable cost and time to study through experiments. For instance, the question “How do atoms arrange themselves at the interface when two solids are brought together?” can be answered relatively easily through a computational approach. In computational materials science, simulations help users evaluate different materials to identify the one(s) with desirable properties. Simulations are also being used to design materials with desired properties. As a result, this field has found applications in a lot of industries, ranging from aerospace to fabrics.
After my PhD and a short postdoctoral stint, I got a research position suited to my background in the semiconductor industry. For the past several decades, Moore’s law, i.e., the doubling of transistor density approximately every 2 years, has been the guiding principle in the semiconductor industry. Of late, materials innovation has played a huge role in helping semiconductor technologists keep up with Moore’s law. My job involves performing simulations to screen materials that could lead to better semiconductors.
There are multiple options in industry for people who want to pursue a career in computational physics research. Physicists are a well-sought-after group, mainly due to their strong mathematical training and analytical skills. A little bit of coding knowledge further enhances one’s job prospects. This does not require much extra effort, as most data collection and analysis work in graduate school involves writing scripts—a great way to hone your coding skills. Across industries, I see an increasing reliance on computer simulations to understand and verify ideas before building actual prototypes, and I think it is a great time to get into the field of computational physics, especially if one is interested in a career outside of academia.

Theoretical physicist Bret Underwood (right) and former student Auberry Fortuner (2013) explore the physics that gave rise to the expansion of the universe. Photo by John Froschauer, Pacific Lutheran University.
by Bret Underwood, Associate Professor at Pacific Lutheran University
“Hmm...What now?” It’s a familiar feeling, being stumped on a physics problem, not sure what to do next. But this was a different type of problem. As a junior physics major, I was strongly considering graduate school in physics. I enjoyed studying physics and had some research experience as an undergraduate. But I was a little intimidated by the graduate school application process. What field should I study?
I read about current research areas on faculty webpages and in journals like Physics Today and realized I was most excited about particle physics and cosmology. However, I was not particularly confident about my laboratory skills (perhaps my instructors felt the same way), so I felt theoretical physics was a better fit. I talked with my faculty mentors about graduate school and the application process, and discussed my sketched-out plans with my fellow students. I learned that graduate school was not just going to be more physics classes, but would also require me to take a much more active role in creating new physics. I thought that sounded pretty cool, so I chose some graduate schools that were doing things I was excited about and submitted my applications.
In graduate school, I began studying problems like a theoretical physicist, refining the same skills I used in my graduate school decision: reading, learning new ideas and techniques, and discussing with others. For example, my first research project involved studying the aftermath of a string theory model of a period of the early universe that underwent a very brief rapid expansion called inflation. In order to understand this model, I had to learn general relativity, string theory, and relativistic field theory, as well as the more specific topics of inflation, D-branes, Kaluza-Klein modes, and postinflationary reheating. If you don’t know what most of these things are, that’s okay—I didn’t either at the time. Stacks of books and journal articles covered my desk as I read, reproduced calculations, discussed with my advisor and other students, and generally tried to figure out what calculations of my own I could do. I might occasionally use a computer program to do some particularly nasty algebra, but mostly I did long calculations that I checked against existing results in the literature and then extended into new results. Eventually, with guidance from my advisor, I found a way to combine these ideas from different areas of physics into a single model.
Today, as a professor at a liberal arts college, I still use the skills of a theoretical physicist, whether I’m working on a research project or revising my teaching. Reading the literature, learning new ideas and methods, and discussing with colleagues are essential techniques for me anytime I’m facing that familiar question: “Hmmm...Now what?”

Medical physicist Jasmine Oliver. Photo courtesy of Jasmine Oliver.
by Jasmine Oliver, Medical Physics Resident at Orlando Health UF Health Cancer Center
I was initially introduced to medical physics at South Carolina State University at a summer orientation for incoming freshman. I was a biology major with plans to attend medical school until a talk on medical physics piqued my interest. I left the orientation and mulled over my options for the remainder of the summer. When I returned in August, I was a physics major. I reasoned that a physics degree could work for either medical school or medical physics, and by the beginning of my senior year at SC State, I’d decided that medical physics was the field for me. Fast-forward through graduate school in medical physics and a postdoctoral fellowship, and now I am a medical physics resident at the Orlando Health UF Health Cancer Center. This is a two-year training position for medical physicists and a requirement for the American Board of Radiology certification in medical physics.
Medical physics falls into the subdiscipline of applied physics. An applied physicist is a physicist who applies physics principles to practical situations. For example, as a medical physicist I apply physics concepts to treat cancer patients with radiation. Applied physics is a bridge between physics and engineering. Applied physics research differs from “pure physics” research because it is being applied in real-time for practical use. “Pure physics” research forms the fundamental basis for applied physics research.
Although the work environment is different, there are many similarities between applied physics and experimental physics. Both are hands-on areas that commonly require scientists to calibrate, operate, and troubleshoot equipment. Both fields value people who can assess data quickly and solve problems creatively. The ability to work well with others on a common goal is essential, whether that be most effectively treating a patient or detecting gravitational waves.
If you are interested in pursuing a career in experimental or applied physics research, here’s some advice:
- Do your research (no pun intended). Google various physics subdisciplines and talk with people in those fields, if possible. Even better, make an effort to shadow these professionals or at least visit their labs. Apply for internships and research experiences in your top fields. Be sure to consider salary, work/life balance, job availability, and location of available positions in your decision-making process.
- Begin developing the necessary skills for your future position now. Take as many math, physics, lab, and related courses as possible. Expose yourself to as much knowledge as possible and look for connections between disciplines. In my experience, I have found that areas of physics where I connect the dots with other science disciplines (e.g., chemistry) are easier to fully comprehend.
- Begin thinking and acting like a scientist/researcher now. Analyze situations and challenge yourself to creatively solve problems using the resources you have on hand. //